Refereed publications in so-called high-impact journals:
- Stute, M., Clement, A., Lohmann, G., 2001: Global climate models: Past, present, and future. Proc. Natl. Acad. Sci. USA, Vol. 98, Issue 19, 10529-10530. (pdf), (Abstract of the talk)
- Knorr, G., and G. Lohmann, 2003: Southern Ocean Origin for Resumption of Atlantic Thermohaline Circulation during Deglaciation. Nature, 424, 532-536. Abstract pdf , link , news and views
- Felis, T., G. Lohmann, H. Kuhnert, S. Lorenz, D. Scholz, J. Pätzold, S. A. Al-Rousan, S. M. Al-Moghrabi, 2004: Increased seasonality in Middle East temperatures during the last interglacial period. Nature 429, 164-168. link Nature-link Nature-highlight
- Drysdale, R.N., J.C. Hellstrom, G. Zanchetta, A.E. Fallick, M.F. Sánchez Gõni, I. Couchoud, J. McDonald, R. Maas, G. Lohmann & I. Isola, 2009: Evidence for obliquity forcing of glacial Termination II. Science 325, 1527-1531. DOI: 10.1126/science.1170371 (link) (pdf)
- Laepple, T., M. Werner, and G. Lohmann, 2011: Synchronicity of Antarctic temperatures and local solar insolation on orbital time-scales. Nature, 471, 91–94. doi:10.1038/nature09825 (link, nv) (press)
- Laepple, T., M. Werner, and G. Lohmann, 2011: Reply on "Antarctic accumulation seasonality". Brief communication arising. Nature 479, E2–E4. doi:10.1038/nature10614 (link) (pdf)
- Salzmann, U., A. M. Dolan, A. M. Haywood, W.-L. Chan, D. J. Hill, A. Abe-Ouchi, B. Otto-Bliesner, F. Bragg, M. A. Chandler, C. Contoux, A. Jost, Y. Kamae, G. Lohmann, D. J. Lunt, S. J. Pickering, M. J. Pound, G. Ramstein, N. A. Rosenbloom, L. Sohl, C. Stepanek, H. Ueda, Z. Zhang, 2013: Challenges in quantifying Pliocene terrestrial warming revealed by data-model discord. Nature Climate Change, 3, 969-974, doi:10.1038/nclimate2008 (link)
- Knorr, G., and G. Lohmann, 2014: A warming climate during the Antarctic ice sheet growth at the Middle Miocene transition. Nature Geoscience, 7, 376–381. doi:10.1038/NGEO2119 (link) (AWI press release) (press release)
- Weber, M. E., Clark, P. U., Kuhn, G., Timmermann, A., Sprenk, D., Gladstone, R., Zhang, X., Lohmann, G., Menviel, L., Chikamoto, M. O., Friedrich, T., Ohlwein, C., 2014: Millennial-scale variability in Antarctic ice-sheet discharge during the last deglaciation. Nature 510, 134–138, doi:10.1038/nature13397 (link) (pdf) (news and views) (press release in German) (press release) (press release AWI)
- Zhang, X., G. Lohmann, G. Knorr, C. Purcell, 2014: Abrupt glacial climate shifts controlled by ice sheet changes. Nature 512, 290–294, DOI: 10.1038/nature13592 (link) (press) (press AWI)
- Liu, Z., J. Zhu, Y. Rosenthal, X. Zhang, B. Otto-Bliesner, A. Timmermann, R.S. Smith, G. Lohmann, W. Zheng, O. E. Timm, 2014: The Holocene temperature conundrum. Proc. Natl. Acad. Sci. USA, vol. 111 no. 34, 3501–3505, doi: 10.1073/pnas.1407229111 (link) (press)
- Mudelsee, M., T. Bickert, C. H. Lear, and G. Lohmann, 2014: Cenozoic climate changes: A review based on time series analysis of marine benthic δ18O records, Rev. Geophys., 52, 333-374, doi:10.1002/2013RG000440. (link) (link2) (data)
- Felis, T., C. Giry, D. Scholz, G. Lohmann, M. Pfeiffer, J. Pätzold, M. Kölling, S. R. Scheffers, 2015: Tropical Atlantic temperature seasonality at the end of the last interglacial. Nature Comm. 6:6159; DOI: 10.1038/ncomms7159 (link)
- Winter A., D. Zanchettin, T. Miller, Y. Kushnir, D. Black, G. Lohmann, A. Burnett, G. Haug, J. Estrella-Martínez, S. F.M. Breitenbach, L. Beaufort, A. Rubino, H. Cheng, 2015: Persistent drying in the tropics linked to natural forcing. Nature comm. 6:7627 doi: 10.1038/ncomms8627 (link)
- Abelmann, A., R. Gersonde, G. Knorr, X. Zhang, B. Chapligin, E. Maier, O. Esper, H. Friedrichsen, G. Lohmann, H. Meyer, and R. Tiedemann, 2015: The seasonal sea ice zone in the glacial Southern Ocean as a carbon sink. Nature comm. 6, 8136. DOI: 10.1038/ncomms9136 (link) (press release AWI German) (press release AWI)
- Stein, R., K. Fahl, M. Schreck, G. Knorr, F. Niessen, M. Forwick, C. Gebhardt, L. Jensen, M. Kaminski, A. Kopf, J. Matthiessen, W. Jokat, and G. Lohmann, 2016: Evidence for ice-free summers in the late Miocene central Arctic Ocean. Nature comm. 7, 11148, doi:10.1038/ncomms11148 (link) ( press release) (German press release) http://scimondo.de/7115/arktis-war-vor-sechs-millionen-jahren-im-sommer-eisfrei/
- Wassenburg, J. A., S. Dietrich, J. Fietzke, J. Fohlmeister, K. P. Jochum, D. Scholz, D. K. Richter, A. Sabaoui, C. Spötl, G. Lohmann, M. O. Andreae, A. Immenhauser, 2016: Major reorganization of the North Atlantic Oscillation during Early Holocene deglaciation. Nature Geo, 9, 602 - 605. doi:10.1038/ngeo2767 (link) (German press release) (press release)
- Staerz, M., W. Jokat, G. Knorr, G. Lohmann, 2017: Threshold in North Atlantic-Arctic Ocean circulation controlled by the subsidence of the Greenland-Scotland Ridge. Nature comm. 8, 15681. DOI: 10.1038/ncomms15681 (link) (press release) (press release German)
- Zhang, X., G. Knorr, G. Lohmann, S. Barker, 2017: Abrupt North Atlantic circulation changes in response to gradual CO2 forcing in a glacial climate state. Nature Geo. DOI: 10.1038/NGEO2974 (link) (press release) (press release German) (press release Cardiff) (press release PalMod)
- Hasenclever, J., G. Knorr, L. Rüpke, P. Köhler, J. Morgan, K. Garofalo, S. Barker, G. Lohmann, I. R. Hall, 2017: Sea level fall during glaciation stabilized atmospheric CO2 by enhanced volcanic degassing. Nature comm. 8, 15867; doi:10.1038/ncomms15867 (link) (press release) (press release German)
- Stein, R., K. Fahl, P. Gierz, F. Niessen, and G. Lohmann, 2017: Arctic Ocean sea ice cover during the penultimate glacial and last interglacial. Nature comm. 8: 373. doi:10.1038/s41467-017-00552-1 (link) (press release) (press release German)
- Lembke-Jene, L., R. Tiedemann, D. Nürnberg, X. Gong, G. Lohmann, 2018: A rapid shift and millennial-scale variations in Holocene North Pacific Intermediate Water ventilation. Proc. Natl. Acad. Sci. 115 (21) 5365-5370. doi:10.1073/pnas.1714754115 (link). (press release German), (press release)
- Colleoni, F., L. De Santis, C. S. Siddoway, A. Bergamasco, N. Golledge, G. Lohmann, S. Passchier, and M. Siegert, 2018: Spatio-temporal variability of processes across Antarctic ice-bed-ocean interfaces. Nature comm. 9: 2289, DOI: 10.1038/s41467-018-04583-0 (link), (pdf), (pdf of the Review Article) (German)
- Maier, E., X. Zhang, A. Abelmann, R. Gersonde, S. Mulitza, M. Werner, M. Méheust, J. Ren, B. Chapligin, H. Meyer, R. Stein, R. Tiedemann, G. Lohmann, 2018: North Pacific freshwater events linked to glacial ocean circulation changes. Nature 559, 241–245. Doi:10.1038/s41586-018-0276-y (news and views) (link) (press release German), (press release)
- Werner, M., J. Jouzel, V. Masson-Delmotte, G. Lohmann, 2018: Reconciling glacial-interglacial changes of Antarctic water stable isotopes, ice sheet topography, and the isotopic paleothermometer. Nature comm. 9, 3537. DOI:10.1038/s41467-018-05430-y (link) (German)
- Gong, X., L. Lembke-Jene, G. Lohmann, G. Knorr, R. Tiedemann, J. Zou, X. Shi, 2019: Enhanced North Pacific deep-ocean stratification by stronger Intermediate water formation during the Heinrich Stadial 1. Nature comm., 10, 656. doi: 10.1038/s41467-019-08606-2 (link) (German)
- Klages, J.P., Salzmann, U., Bickert, T., Hillenbrand, C.-D., Gohl, K., Kuhn, G., Bohaty, S., Titschack, J., Müller, J., Frederichs, T., Bauersachs, T., Ehrmann, W., van de Flierdt, T., Simões Pereira, P., Larter, R.D., Lohmann, G., Niezgodzki, I., Uenzelmann-Neben, G., Zundel, M., Spiegel, C., Francis, J.E., Nehrke, G., Schwarz, F., Smith, J.A., Freudenthal, T., Esper, O., Pälike, H., Ronge, T., Dziadek, R., and the Science Team of Expedition PS104, 2020: Temperate rainforests near the South Pole during peak Cretaceous warmth. Nature, 580, 81–86. doi:10.1038/s41586-020-2148-5 (link) (cover) (data) (model output) (press)
- Wang, X., B. Carrapa, Y. Sun, D. Dettman, J. Chapman, J. C. Rugenstein, M. Clementz, P. DeCelles, M. Wang, J. Chen, J. Quade, F. Wang, Z. Li, I. Oimuhammadzoda, M. Gadoev, X. Zhang, G. Lohmann, and F. Chen, 2020: The role of the westerlies and orography on Asian hydroclimate since the Late Oligocene. Geology 48 (7), 728-732. doi:10.1130/G47400.1 (link)
- Gowan, E.J., X. Zhang, S. Khosravi, A. Rovere, P. Stocchi, A. L. C. Hughes, R. Gyllencreutz, J. Mangerud, J. I. Svendsen, G. Lohmann, 2021: A new global ice sheet reconstruction for the past 80,000 years. Nature comm. 12, 1199. https://doi.org/10.1038/s41467-021-21469-w, data (press release German) (press release English)
- Zhang, X., S. Barker, G. Knorr, G. Lohmann, Y. Sun, R. Drysdale, D. Hodell, F. Chen, 2021: Direct astronomical influence on abrupt climate variability. Nature Geo. 14, pages 819–826. doi:10.1038/s41561-021-00846-6 (link) (pdf) (press)
- Knorr, G., S. Barker, X. Zhang, G. Lohmann, X. Gong, P. Gierz, C. Stepanek, L. Stap, 2021: A salty deep ocean as a prerequisite for glacial termination. Nature Geo, 14, 930–936. doi:10.1038/s41561-021-00857-3 (link) (press) (press German)
- Feng, R., T. Bhattacharya, B. Otto-Bliesner, E. Brady, A. Haywood , J. Tindall, S. Hunter, A. Abe-Ouchi, W-L Chan, C. Contoux, C. Guo, X. Li, G. Lohmann, C. Stepanek, N. Tan, Q. Zhang, Z. Zhang, Z.Han, J. R. Williams, D. J. Lunt, H. Dowsett, D. Chandan, W. R. Peltier, 2022: Past terrestrial hydroclimate sensitivity controlled by Earth System Feedbacks. Nature Communications. 13: 1306 https://doi.org/10.1038/s41467-022-28814-7
- Pontes, G., A. Taschetto, A. Sen Gupta, A. Santoso, I. Wainer, A. Haywood, W.-L. Chan, A. Abe-Ouchi, C. Stepanek, G. Lohmann, S. Hunter, J. Tindall, M. Chandler, L. Sohl, D. Peltier, D. Chandan, Y. Kamae, K. Nisancioglu, Z. Zhang, C. Contoux, N. Tan, Q. Zhang, B. Otto-Bliesner, E. Brady, R. Feng, A. von der Heydt, M. Baatsen, A. Oldemann, 2022: Northward ITCZ shift drives reduced ENSO activity in the Mid-Pliocene Warm Period. Nature Geoscience DOI:10.1038/s41561-022-00999-y https://www.nature.com/articles/s41561-022-00999-y (Info) (press)
- Dong, J., X. Shi, X. Gong, Astakhov, A.S., Hu, L., Liu, X., Yang, G., Wang, Y., Vasilenko, Y., Qiao, S., Bosin, A., G. Lohmann, 2022: Enhanced Arctic sea ice melting controlled by larger heat discharge of Holocene rivers. Nature comm. 13, 5368. https://doi.org/10.1038/s41467-022-33106-1
- Gowan, E.J., X. Zhang, S. Khosravi, A. Rovere, P. Stocchi, A. L. C. Hughes, R. Gyllencreutz, J. Mangerud, J. I. Svendsen, G. Lohmann, 2022: Reply to: Towards solving the missing ice problem and the importance of rigorous model data comparisons. Nature comm. 13, 6264, doi:10.1038/s41467-022-33954-x
- Hörhold, M., Münch, T., Weißbach S., Kipfstuhl S., Freitag J., Sasgen I., Lohmann G., Vinther B. and T. Laepple, 2023: Exceptional temperatures in central-north Greenland ice cores. Nature, 613, 503–507. doi:10.1038/s41586-022-05517-z https://www.nature.com/articles/s41586-022-05517-z (press German) (press)
- Guo, D., H Wang, V Romanovsky, A Haywood, N Pepin, J Sun, Q Yan, Z Zhang, X Li, B Otto-Bliesner, R Feng, G Lohmann, C Stepanek, A Abe-Ouchi, W-L Chan, W. R. Peltier, D. Chandan, A. von der Heydt, C. Contoux, M. Chandler, N Tan, Q Zhang, S Hunter, Y Kamae 2023: Highly restricted near-surface permafrost extent during the mid-Pliocene warm period. Proceedings of the National Academy of Sciences, 120 (36) e2301954120 doi:10.1073/pnas.2301954120 (press German) (press English)
- Jonkers, L., T. Laepple, M. C. Rillo, X. Shi, A. M. Dolman, G. Lohmann, A. Paul, A. Mix, and M. Kucera, 2023: Plankton biogeography shows strong meridional variation in ice age to modern ocean warming. Nat. Geosci., DOI: 10.1038/s41561-023-01328-7 (link) (news and views) (press)
- Niu, L., G. Knorr, U. Krebs-Kanzow, P. Gierz, G. Lohmann, 2024: Rapid Laurentide Ice Sheet growth preceding the Last Glacial Maximum due to summer snowfall. Nat Geo., DOI:10.1038/s41561-024-01419-z https://www.nature.com/articles/s41561-024-01419-z (press) (press German)
- Nitzbon, J., T. Schneider von Deimling, M. Aliyeva, S. E. Chadburn, G. Grosse, S. Laboor, H. Lee, G. Lohmann, N. Steinert, S. Stuenzi, M. Werner, S. Westermann, M. Langer, 2024: No respite from permafrost-thaw impacts in absence of a global tipping point. Nature Climate Change https://www.nature.com/articles/s41558-024-02011-4 , (press)
- Klages, J.P., C.-D. Hillenbrand, S. M. Bohaty, U. Salzmann, T. Bickert, G. Lohmann, H. S. Knahl, P. Gierz, L. Niu, J. Titschack, G. Kuhn, T. Frederichs, J. Müller, T. Bauersachs, R. D. Larter, K. Hochmuth, W. Ehrmann, G. Nehrke, F. J. Rodríguez-Tovar, G. Schmiedl, S. Spezzaferri, A. Läufer, F. Lisker, T. van de Flierdt, A. Eisenhauer, G. Uenzelmann-Neben, O. Esper, J. A. Smith, H. Pälike, C. Spiegel, R. Dziadek, T. A. Ronge, T. Freudenthal, and K. Gohl, 2024: Ice sheet-free West Antarctica during peak early Oligocene glaciation. Science, 385, 322-327. doi:10.1126/science.adj3931 (Press German) (Geburtsstunde pro-physik) (Press) (Film at 16:42) (Info) (pdf)
- Gou, R., G. Lohmann, L. Wu, 2024: Atlantic meridional overturning circulation decline: Tipping small scales under global warming. Physical Review Letters, 133, 034201, doi:10.1103/PhysRevLett.133.034201 (link) (link to research news) (link to research news, "The major Atlantic current that keeps Northern Europe warm could have new variations and tipping points") (press) (German press) (pdf)
- An, Z., W Zhou, Z Zhang, X Zhang, Z Liu, Y Sun, S C. Clemens, L Wu, J Zhao, Z Shi, X Ma, H Yan, G Li, Y Cai, J Yu, Y Sun, S Li, Y Zhang, C Stepanek, G Lohmann, G Dong, H Cheng, Y Liu, Z Jin, T Li, Y Hao, J Lei, W Cai, 2024: Mid-Pleistocene climate transition triggered by Antarctic ice-sheet growth. Science, 385, 560-565. DOI: 10.1126/science.abn4861 (link) (German press) (press)
- Akabane TK, Chiessi CM, Hirota M, Bouimetarhan I, Prange M, Mulitza S, Bertassoli Jr. DJ, Häggi C, Staal A, Lohmann G, Boers N, Daniau AL, Oliveira RS, Campos MC, Shi X, De Oliveira PE, 2014: Slowdown of ocean circulation increases the vulnerability of northern Amazon forests. Nature Geoscience 2024. DOI: 10.1038/s41561-024-01578-z (link) (press) (German press)
- Ma, Q., X Shi, D Sidorenko, P Scholz, G Lohmann, M Ionita, 2024: Revisiting climate impacts of an AMOC slowdown: dependence on freshwater locations in the North Atlantic. Science Advances 10,eadr3243(2024).DOI:10.1126/sciadv.adr3243 (link) (press)
- Wirtz, K., N Antunes, A Diachenko, J Laabs, C Lemmen, G Lohmann, R McLaughlin, E Zorita-Calvo, D Gronenborn, 2024: Multicentennial cycles in continental demography synchronous with solar activity and climate stability. Nat Commun 15, 10248 (2024). https://doi.org/10.1038/s41467-024-54474-w
- Gou, R., K. Wolf, C. Hoppe, L. Wu, G. Lohmann, 2025: The changing nature of future Arctic marine heatwaves and its potential impacts on the ecosystem. Nature Climate Change, 15, 162–170, https://doi.org/10.1038/s41558-024-02224-7 (press) (German press)
- Song, P., P. Scholz, G. Knorr, D. Sidorenko, R. Timmermann, G. Lohmann, 2025: Thresholds of accelerated Antarctic basal melt in climate projections. Nat. Clim. Chang. (2025). https://doi.org/10.1038/s41558-025-02306-0
Books:
- Lohmann, G., 1996: Stability of the thermohaline circulation in analytical and numerical models, Ph. D. Dissertation, University of Bremen (Germany), 128 pp. [ Reports on Polar Research 200, Alfred Wegener Institute Bremerhaven]. doi:10.2312/BzP_0200_1996 Abstract/Zusammenfassung
- Fischer, H., Kumke, T., Lohmann, G., Flöser, G., Miller, H., Storch, H.v., Negendank, J.F.W., 2004: Introduction to the KIHZ project, pp. v-vii. In: The climate in historical times: Toward a synthesis of Holocene proxy data and climate models, Springer-Verlag, Berlin Heidelberg New York. Fischer, H.; Kumke, T.; Lohmann, G.; Flöser, G.; Miller, H.; Storch, H.v.; Negendank, J.F.W. (Eds.) link
- Lohmann, G., R. Treffeisen, A. Wagner, A. Kornmann, M. Weigelt, 2013a. Naturwissenschaftliche Perspektive der Klimawissenschaft 1, M12. Fernuniversität in Hagen. Umweltwissenschaften (infernum) der Fernuniversität Hagen link
- Lohmann, G., R. Treffeisen, A. Wagner, A. Kornmann, M. Weigelt, 2013b. Naturwissenschaftliche Perspektive der Klimawissenschaft 2, M12. Fernuniversität in Hagen. Umweltwissenschaften (infernum) der Fernuniversität Hagen link
- Lohmann, G., K. Grosfeld, D. Wolf-Gladrow, V. Unitan, J. Notholt, and A. Wegener (Eds.), 2013: "Earth System Science: Bridging the Gaps between Disciplines. Perspectives from a Multi-disciplinary Helmholtz Research School". Series: SpringerBriefs in Earth System Sciences, 2013, 138 p. 61 illus., 52 in color. ISBN: 978-3-642-32234-1 (Print) 978-3-642-32235-8 (Online) Springer, Heidelberg. doi: 10.1007/978-3-642-32235-8; link link2
- Chirila, D., and G. Lohmann, 2015: Introduction to Modern Fortran for Earth System Sciences. 2015, XXII, 250 pages. 15 illus., 10 illus. in color. ISBN: 978-3-642-37008-3; Springer, Berlin Heidelberg. link
- Lohmann, G., H. Meggers, V. Unnithan, D. Wolf-Gladrow, J. Notholt, A. Bracher (eds.), Towards an Interdisciplinary Approach in Earth System Science: Advances of a Helmholtz Research School. Springer Earth System Sciences, Heidelberg, Germany. 2015, X, 251 pages, 83 illus. in color. ISBN 978-3-319-13864-0. doi:10.1007/978-3-319-13865-7 (link)
- Lohmann, G., R. Treffeisen, K. Grosfeld, C. Danek, 2019a. Naturwissenschaftliche Perspektive der Klimawissenschaft 1, M12. Fernuniversität in Hagen. Umweltwissenschaften (infernum) der Fernuniversität Hagen ( link ) ( link FU Hagen)
- Lohmann, G., R. Treffeisen, K. Grosfeld, C. Danek, 2019b. Naturwissenschaftliche Perspektive der Klimawissenschaft 2, M12. Fernuniversität in Hagen. Umweltwissenschaften (infernum) der Fernuniversität Hagen link
- Conversations on Climate: The People Behind the Science. Lohmann, G., (Ed.), 2025: SpringerBriefs in Climate Studies. 978-3-031-81649-9 (ISBN), pp. 118 (link AWI) ; (link Springer) ; webinar
- Klimagespräche. Interviews mit Klimawissenschaftler*innen. Lohmann, G., (Ed.), 2025. Springer (link) Softcover ISBN 978-3-662-70419-6, eBook ISBN 978-3-662-70420-2 (pdf) Erscheint Mai 2025.
Colleoni, F., L. De Santis, C. S. Siddoway, A. Bergamasco, N. Golledge, G. Lohmann, S. Passchier, and M. Siegert, 2018:
Spatio-temporal variability of processes across Antarctic ice-bed-ocean interfaces.
Nature comm. 9: 2289,
DOI: 10.1038/s41467-018-04583-0
(link), (pdf),
(pdf of the Review Article)
(German)
Werner, M., J. Jouzel, V. Masson-Delmotte, G. Lohmann, 2018:
Reconciling glacial-interglacial changes of Antarctic water stable isotopes,
ice sheet topography, and the isotopic paleothermometer.
Nature comm. 9, 3537.
DOI:10.1038/s41467-018-05430-y
(link)
(German)
Gong, X., L. Lembke-Jene, G. Lohmann, G. Knorr, R. Tiedemann, J. Zou, X. Shi, 2019:
Enhanced North Pacific deep-ocean stratification by stronger Intermediate water formation during the Heinrich Stadial 1. Nature comm., 10, 656. doi: 10.1038/s41467-019-08606-2
(link)
(German)
Zhang, X., S. Barker, G. Knorr, G. Lohmann, Y. Sun, R. Drysdale, D. Hodell, F. Chen, 2021:
Direct astronomical influence on abrupt climate variability. Nature Geo.
doi:10.1038/s41561-021-00846-6
(link)
(pdf)
(press)
A new study by Knorr et al. study provides a solution to this problem. With the aid of climate model simulations Knorr et al. show that a build-up of relatively warm (although still cold) and salty waters in the abyssal ocean during full glacial conditions might be responsible for the exaggerated warming observed across Antarctica associated with deglacial shifts in ocean circulation. When Atlantic Ocean circulation weakens abruptly, cold abyssal waters around Antarctica are preferentially transported upwards towards the surface where they reduce the tendency for surface warming to occur in response to the weakened circulation and associated reduction in the northward oceanic heat transport. If the deep waters are warmer, then the cooling effect will be reduced, allowing a greater degree of warming to occur at the surface and across Antarctica. Crucially for this mechanism to work, glacial deep waters must also be saltier, in order to offset the loss of density due to their warmer temperature. According to the new results, the mechanism can produce a doubling in the net rate of warming across Antarctica on a multi-centennial timescale. The authors argue that this helps to explain the enhanced warming observed during deglacial transitions. Therefore, they argue, deglacial shifts in ocean circulation are special because they tap into deep waters that are on average warmer (relative to intermediate depths) and saltier than at any other time during a glacial cycle.
Figure
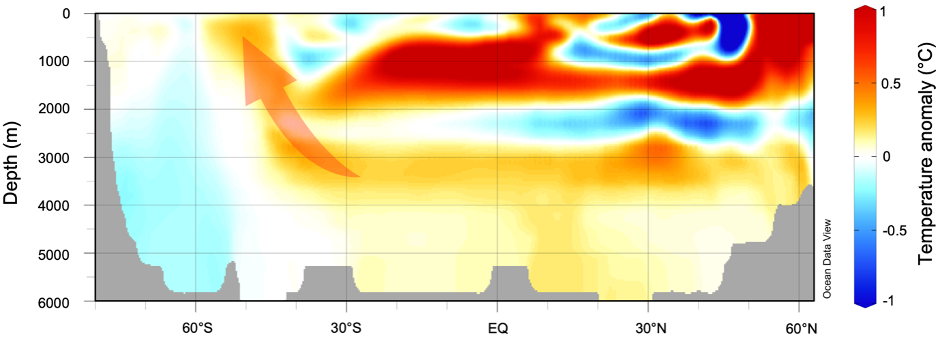
Temperature difference between the glacial and interglacial anomalies in response to an AMOC weakening. Shown are conditions between model years 100 and 200 as a 100 year mean, zonally averaged in the Atlantic sector (modified from Fig. 5 in Knorr et al., 2021).
Knorr, G., Barker, S., Zhang, X., Lohmann, G., Gong, G., Gierz, P., Stepanek, C., L. B. Stap: A salty deep ocean as a prerequisite for glacial termination. Nature Geoscience 14, 930–936 (2021). https://doi.org/10.1038/s41561-021-00857-3
Knorr, G., Barker, S., Zhang, X., Lohmann, G., Gong, G., Gierz, P., Stepanek, C., L. B. Stap: A salty deep ocean as a prerequisite for glacial termination. Nature Geoscience 14, 930–936 (2021). https://doi.org/10.1038/s41561-021-00857-3
Abbildung
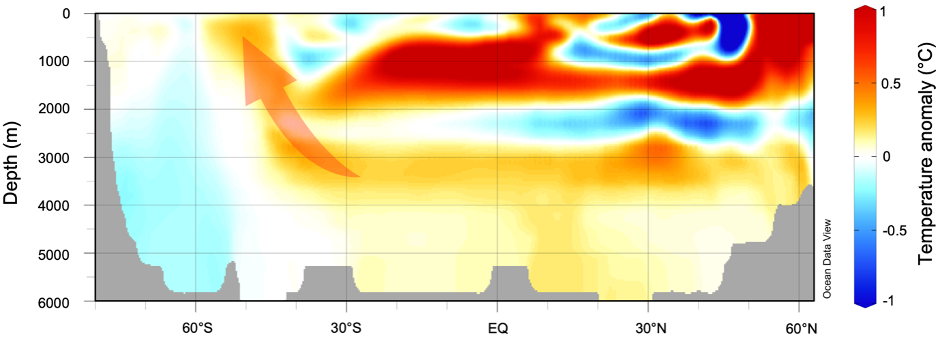
Differenz zwischen glazialen und interglazialen Temperatur-Anomalien die als Reaktion auf eine Abschwächung der AMOC im Ozean auftreten. Gezeigt werden hier Bedingungen zwischen den Modelljahren 100 und 200 als Mittel über 100 Jahre, zonal gemittelt über den atlantischen Sektor (angepasste Version der Abbildung 5 des Artikels von Knorr et al., 2021).
Knorr, G., Barker, S., Zhang, X., Lohmann, G., Gong, G., Gierz, P., Stepanek, C., L. B. Stap: A salty deep ocean as a prerequisite for glacial termination. Nature Geoscience 14, 930–936 (2021). https://doi.org/10.1038/s41561-021-00857-3
Die warmen (El Niño) und kalten (La Niña) Ereignisse verursachen erhebliche Veränderungen der Wettermuster und der Ozeanzirkulation, was sich auf die Landwirtschaft, die Fischerei,
und eine Vielzahl anderer Phänomene auswirkt. Angesichts seiner ausgeprägten sozioökonomischen
Auswirkungen und der potenziellen Vorhersagbarkeit wird El Niño - Southern Oscillation (ENSO) intensiv erforscht. Ob und wie sich ENSO in Abhängigkeit von Treibhausgasen und anderen Einflüsse verändert, kann durch die Untersuchung des vergangenen, gegenwärtigen und zukünftigen Klimas mit Hilfe von Paläo-Rekonstruktionen, instrumentellen Aufzeichnungen, Theorie und numerischen Simulationen untersucht werden.
Speziell geht um die Frage der Eigenschaften von ENSO in einem wärmeren Klima, dem mittleren Pliozän, welches als ein potentielles Analogon für zukünftige warme Klimata betrachtet werden kann. Dieses wird in einem internationalen Modellvergleichsprojekt, bei dem das AWI seit Jahren aktiv beteiligt ist, untersucht. Die Modelle zeigen, dass ENSO im wärmeren Klima schwächer ist, und dass zur Erklärung dieses Verhaltens die nordwärtige Verschiebung der Inner-Tropischen Konvergenz Zone (engl. ITCZ) berücksichtigt werden muss. Vergangene und zukünftige Änderungen in ENSO stehen in direktem Zusammenhang mit der klimatologischen Position der ITCZ.
Pontes, G., A. Taschetto, A. Sen Gupta, A. Santoso, I. Wainer, A. Haywood, W.-L. Chan, A. Abe-Ouchi, C. Stepanek, G. Lohmann, S. Hunter, J. Tindall, M. Chandler, L. Sohl, D. Peltier, D. Chandan, Y. Kamae, K. Nisancioglu, Z. Zhang, C. Contoux, N. Tan, Q. Zhang, B. Otto-Bliesner, E. Brady, R. Feng, A. von der Heydt, M. Baatsen, A. Oldemann, 2022: Northward ITCZ shift drives reduced ENSO activity in the Mid-Pliocene Warm Period. Nature Geoscience DOI:10.1038/s41561-022-00999-y https://www.nature.com/articles/s41561-022-00999-y
In recent years global warming has left its mark on the Antarctic ice sheets. The "eternal" ice in Antarctica is melting faster than previously assumed, particularly in West Antarctica more than East Antarctica. The root for this could lie in its formation, as an international research team led by the Alfred Wegener Institute has now discovered: sediment samples from drill cores combined with complex climate and ice-sheet modelling show that permanent glaciation of Antarctica began around 34 million years ago – but did not encompass the entire continent as previously assumed, but rather was confined to the eastern region of the continent (East Antarctica). It was not until at least 7 million years later that ice was able to advance towards West Antarctic coasts. The results of the new study show how substantially differently East and West Antarctica react to external forcing, as the researchers describe in the prestigious journal Science.
Around 34 million years ago, our planet underwent one of the most fundamental climate shifts that still influences global climate conditions today: the transition from a greenhouse world, with no or very little accumulation of continental ice, to an icehouse world, with large permanently glaciated areas. During this time, the Antarctic ice sheet built up. How, when and, above all, where, was not yet known due to a lack of reliable data and samples from key regions, especially from West Antarctica, that document the changes in the past.
Based on a drill core that the researchers retrieved using the MARUM-MeBo70 seafloor drill rig in a location offshore the Pine Island Glacier on the Amundsen Sea coast of West Antarctica, they were able to establish the history of the dawn of the icy Antarctic continent for the first time. Surprisingly, no signs of the presence of ice can be found in this region during the first major phase of Antarctic glaciation. “This means that a large-scale, permanent first glaciation must have begun somewhere in East Antarctica,” says Dr Johann Klages, geologist at the AWI who led the research team. This is because West Antarctica remained ice-free during this first glacial maximum. At this time, it was still largely covered by dense broadleaf forests and a cool-temperate climate that prevented ice from forming in West Antarctica.
East and West Antarctica react very different to external conditions
In order to better understand where the first permanent ice formed in Antarctica, the AWI palaeoclimate modelers combined the newly available data together with existing data on air and water temperatures and the occurrence of ice. “The simulation has supported the results of the geologists' unique core,” says Prof Dr Gerrit Lohmann, paleoclimate modeler at the AWI. “This completely changes what we know about the first Antarctic glaciation.” According to the study, the basic climatic conditions for the formation of permanent ice only prevailed in the coastal regions of the East Antarctic Northern Victoria Land. Here, moist air masses reached the strongly rising Transantarctic Mountains – ideal conditions for permanent snow and subsequent formation of ice caps. From there, the ice sheet spread rapidly into the East Antarctic hinterland. However, it took some time before it reached West Antarctica: “It wasn't until about seven million years later that conditions allowed for advance of an ice sheet to the West Antarctic coast,” explains Hanna Knahl, a palaeoclimate modeler at the AWI. “Our results clearly show how cold it had to get before the ice could advance to cover West Antarctica that, at that time, was already below sea level in many parts.” What the investigations also impressively show is how different the two regions of the Antarctic ice sheet react to external influences and fundamental climatic changes. “Even a slight warming is enough to cause the ice in West Antarctica to melt again - and that's exactly where we are right now,” adds Johann Klages.
The findings of the international research team are critical for understanding the extreme climate transition from the greenhouse climate to our current icehouse climate. Importantly, the study also provides new insight that allows climate models to simulate more accurately how permanently glaciated areas affect global climate dynamics, that is the interactions between ice, ocean and atmosphere. This is of crucial importance, as Johann Klages says: “Especially in light of the fact that we could be facing such a fundamental climate change again in the near future.”
Using new technology to gain unique insights
The researchers were able to close this knowledge gap with the help of a unique drill core that they retrieved during the expedition PS104 on the research vessel Polarstern in the West Antarctic in 2017. The MARUM-MeBo70 drilling rig developed at MARUM in Bremen was used for the first time in the Antarctic. The seabed off the West Antarctic Pine Island Glacier is so condensed that it was previously impossible to reach deep sediments using conventional drilling methods. The MARUM-MeBo70 has a rotating cutterhead, which made it possible to drill about 10 meters into the seabed and retrieve the samples.
Further material:
More information, including a copy of the paper and pictures, can be found online at the Science press package at .
https://www.eurekalert.org/press/scipak/
Original publication:
J. P. Klages, C.-D. Hillenbrand, S. M. Bohaty, U. Salzmann, T. Bickert, G. Lohmann, H. S. Knahl, P. Gierz, L. Niu, J. Titschack, G. Kuhn, T. Frederichs, J. Müller, T. Bauersachs, R. D. Larter, K. Hochmuth, W. Ehrmann, G. Nehrke, F. J. Rodríguez-Tovar, G. Schmiedl, S. Spezzaferri, A. Läufer, F. Lisker, T. van de Flierdt, A. Eisenhauer, G. Uenzelmann-Neben, O. Esper, J. A. Smith, H. Pälike, C. Spiegel, R. Dziadek, T. A. Ronge, T. Freudenthal, and K. Gohl. Ice sheet-free West Antarctica during peak early Oligocene glaciation. (2024). 10.1126/science.adj3931. This paper will be published online by the journal Science on THURSDAY, 04 July 2024.
Zahlen aus epic für Topic 2: 422